Submitted by taire on
For a cell to migrate, protrusion of the front and translocation of the cell body must be followed by retraction of the rear of the cell. Cell body translocation immediately follows protrusion and is independent of actin polymerization[1]. In keratocytes, the cell body 'rolls' behind the front protrusion[1]. This movement is propelled by a coordinated contraction of the actomyosin cytoskeleton, and thus depends on myosin II[2]. Myosin-mediated contraction and microtubule motors (e.g. dynein) also control the translocation of the nucleus[3],[4]. On the other hand, rear retraction requires the coordinated contraction of the actin cytoskeleton and disassembly of the adhesions at the trailing edge. Several mechanisms converge to promote adhesion disassembly: actomyosin contraction, microtubule-induced adhesion relaxation, endocytosis of adhesion receptors, and proteolytic cleavage of focal adhesion proteins[5],[6],[7],[8].
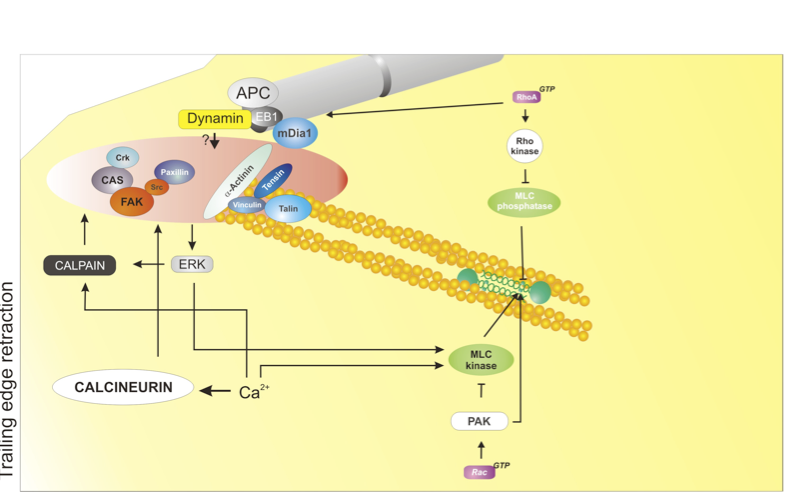
At the rear of the cell, adhesions disassemble; this process is mediated by at least four major mechanisms: 1) actomyosin contraction, controlled by RhoA - Rho-kinase - Myosin II; 2) protein dephosphorylation and inactivation, mediated by phosphatases, e.g. calcineurin; 3) proteolysis of adhesion components, mediated by calpain; 4) microtubule targeting; the identity of the disassembly signal is not known. Used with permission from Vicente-Manzanares et al., 2005 J.Cell Sci. 118,4,4917.
click to enlarge
Retraction
Actomyosin contraction: The actomyosin cytoskeleton (mediated predominantly by myosin II) promotes retraction of the rear of migrating cells[9],[10]. The myosin IIA isoform acts downstream of the RhoA - ROCKaxis and mediates retraction and adhesion disassembly in a variety of cell lines[9],[6],[11],[12],[13],[14],[15].
Microtubule-induced adhesion relaxation: Some adhesions are repeatedly targeted by microtubules - in particular, adhesions at the trailing edge [16]. In many cases, microtubule targeting promotes adhesion disassembly[7]. Dynamin and FAK are required for adhesion disassembly[17]; but whether they mediate endocytosis only (see below) or deliver additional relaxation signal(s) is still unknown.
Endocytosis of adhesion receptors: Emerging evidence points to the endocytosis and recycling of integrins and other receptors, such as GPCRs, as a key mechanism in regulating adhesion turnover[3] and, therefore, as an important step in controlling cell migration. During neutrophil migration, endocytosis drives the internalization and recycling of α5β3 integrin[19]. More specifically, clathrin-mediated endocytosis has been shown to mediate disassembly of a specific subset of adhesions, near the nucleus, by promoting internalization and recycling of β integrins downstream of microtubules, FAK and dynamin[5], and is likely involved in adhesion disassembly during cell migration. On the other hand, integrin recycling - for example by phosphoinositide-dependent kinase-1 (PDK1) and and Rab proteins[20],[21],[22], - plays a key role in directional migration.
Calpain-dependent cleavage: The calpain family of proteases is involved in retraction of the trailing edge of migrating cells[23]. Several proteins that are present in cell-matrix adhesions have been identified as targets of these calcium-dependent enzymes. Cleavage of two of these, talin and FAK, is critical for retraction of the rear of migrating cells[8],[24].
Box: Small Rho GTPases
RhoA was originally described as a serum-responsive element that mediated the formation of stress fibers in adherent cells[25]. RhoA is a key mediator of actin polymerization and reorganization in response to extracellular signals. Multiple GEFs activate RhoA; likewise, multiple GAPs target RhoA. Rho is critically regulated through sequestration in the cellular cytoplasm by RhoGDIs. RhoA can activate different effectors, including: p160ROCK/Rho-kinase, which activates myosin II through direct phosphorylation of RLC[26] and phosphorylation and inactivation of the MLC phosphatase[27], resulting in actin organization into large filament bundles[9]; mDia1, a formin that promotes actin polymerization[28],[29] and also controls microtubule dynamics[30],[31]; citron-K, a kinase that controls RLC phosphorylation and cytokinesis[32]; and others.
Rac was identified as the key mediator in the membrane ruffling response to growth factors; soon, it was revealed that Rac controls protrusion formation[33]. Many GEFs activate Rac: some notable ones are α/β-PIX, Tiam1, DOCK180 and some members of the Vav subfamily. Similarly, multiple GAPs downregulate Rac activity. Rac also activates several specific downstream effectors; for example, PAKs[34], which are serine/threonine kinases that phosphorylate a plethora of substrates implicated in cell migration, including MLCK (inhibitory)[35], RLC (activating)[36], LIMK (activating, which inhibits cofilin, regulating its actin-severing activity)[37], and stathmin (which controls microtubule stability)[38]. Perhaps the best-studied effect of Rac is the dramatic induction of actin polymerization and the formation of dendritic (branched) actin[39]. Rac activates the scaffold protein WAVE/Scar, which in turn activates the Arp2/3 complex, a potent actin nucleator ([40], see Protrusion section).
Cdc42 is a critical regulator of polarity and also drives actin polymerization. The polarity function is conserved from very simple eukaryotes, such as fission or budding yeast, to specialized mammalian cells, such as neurons or leukocytes. An early insight into its function revealed that Cdc42 activation promotes filopodia formation[41]. At this point, it remains unclear whether Cdc42 is sequestered in the cytoplasm by GDIs. Key effectors include: PAK (see above)[42]; the WASP/N-WASP proteins[43], which activate the Arp2/3 complex similar to WAVE/Scar[44]; IQGAP, through which Cdc42 controls actin and microtubules[45]; MRCK, which activates myosin II and controls nuclear repositioning during cell migration[3],[46]; and PAR proteins, in conjunction with aPKC, which also control centrosome reorientation and microtubule polarization through their effect on GSK-3β and APC[47].
- Anderson, K. I., Wang, Y. L. & Small, J. V. Coordination of protrusion and translocation of the keratocyte involves rolling of the cell body. J Cell Biol 134, 1209-18 (1996). Pubmed abstract # 8794862
- Svitkina, T. M., Verkhovsky, A. B., McQuade, K. M. & Borisy, G. G. Analysis of the actin-myosin II system in fish epidermal keratocytes: mechanism of cell body translocation. J Cell Biol 139, 397-415 (1997). Pubmed abstract # 9334344
- Gomes, E. R., Jani, S. & Gundersen, G. G. Nuclear movement regulated by Cdc42, MRCK, myosin, and actin flow establishes MTOC polarization in migrating cells. Cell 121, 451-63 (2005). Pubmed abstract # 15882626
- Levy, J. R. & Holzbaur, E. L. Dynein drives nuclear rotation during forward progression of motile fibroblasts. J Cell Sci 121, 3187-95 (2008). Pubmed abstract # 18782860
- Ezratty, E. J., Bertaux, C., Marcantonio, E. E. & Gundersen, G. G. Clathrin mediates integrin endocytosis for focal adhesion disassembly in migrating cells. J Cell Biol 187, 733-47 (2009). Pubmed abstract # 19951918
- Worthylake, R. A., Lemoine, S., Watson, J. M. & Burridge, K. RhoA is required for monocyte tail retraction during transendothelial migration. J Cell Biol 154, 147-60 (2001). Pubmed abstract # 11448997
- Kaverina, I., Krylyshkina, O. & Small, J. V. Microtubule targeting of substrate contacts promotes their relaxation and dissociation. J Cell Biol 146, 1033-44 (1999). Pubmed abstract # 10477757
- Franco, S. J. et al. Calpain-mediated proteolysis of talin regulates adhesion dynamics. Nat Cell Biol 6, 977-83 (2004).Pubmed abstract # 15448700
- Chrzanowska-Wodnicka, M. & Burridge, K. Rho-stimulated contractility drives the formation of stress fibers and focal adhesions. J Cell Biol 133, 1403-15 (1996). Pubmed abstract # 8682874
- Crowley, E. & Horwitz, A. F. Tyrosine phosphorylation and cytoskeletal tension regulate the release of fibroblast adhesions. J Cell Biol 131, 525-37 (1995). Pubmed abstract # 7593176
- Vicente-Manzanares, M., Zareno, J., Whitmore, L., Choi, C. K. & Horwitz, A. F. Regulation of protrusion, adhesion dynamics, and polarity by myosins IIA and IIB in migrating cells. J Cell Biol 176, 573-80 (2007). Pubmed abstract # 17312025
- Even-Ram, S. et al. Myosin IIA regulates cell motility and actomyosin-microtubule crosstalk. Nat Cell Biol 9, 299-309 (2007). Pubmed abstract # 17310241
- Vicente-Manzanares, M. et al. A role for the Rho-p160 Rho coiled-coil kinase axis in the chemokine stromal cell-derived factor-1alpha-induced lymphocyte actomyosin and microtubular organization and chemotaxis. J Immunol 168, 400-10 (2002). Pubmed abstract # 11751986
- Smith, A., Bracke, M., Leitinger, B., Porter, J. C. & Hogg, N. LFA-1-induced T cell migration on ICAM-1 involves regulation of MLCK-mediated attachment and ROCK-dependent detachment. J Cell Sci 116, 3123-33 (2003). Pubmed abstract # 12799414
- Eddy, R. J., Pierini, L. M., Matsumura, F. & Maxfield, F. R. Ca2+-dependent myosin II activation is required for uropod retraction during neutrophil migration. J Cell Sci 113 ( Pt 7), 1287-98 (2000). Pubmed abstract # 10704379
- Efimov, A. & Kaverina, I. Significance of microtubule catastrophes at focal adhesion sites. Cell Adh Migr 3 285-7 (2009).Pubmed abstract # 19483470
- Ezratty, E. J., Partridge, M. A. & Gundersen, G. G. Microtubule-induced focal adhesion disassembly is mediated by dynamin and focal adhesion kinase. Nat Cell Biol 7, 581-90 (2005). Pubmed abstract # 15895076
- Caswell, P. T., Vadrevu, S. & Norman, J. C. Integrins: masters and slaves of endocytic transport. Nat Rev Mol Cell Biol 10, 843-53 (2009). Pubmed abstract # 19904298
- Pierini, L. M., Lawson, M. A., Eddy, R. J., Hendey, B. & Maxfield, F. R. Oriented endocytic recycling of alpha5beta1 in motile neutrophils. Blood 95, 2471-80 (2000). Pubmed abstract # 10753823
- White, D. P., Caswell, P. T. & Norman, J. C. alpha v beta3 and alpha5beta1 integrin recycling pathways dictate downstream Rho kinase signaling to regulate persistent cell migration. J Cell Biol 177, 515-25 (2007). Pubmed abstract # 17485491
- Caswell, P. T. et al. Rab-coupling protein coordinates recycling of alpha5beta1 integrin and EGFR1 to promote cell migration in 3D microenvironments. J Cell Biol 183, 143-55 (2008). Pubmed abstract # 18838556
- Pellinen, T. et al. Integrin trafficking regulated by Rab21 is necessary for cytokinesis. Dev Cell 15, 371-85 (2008).Pubmed abstract # 18804435
- Huttenlocher, A. et al. Regulation of cell migration by the calcium-dependent protease calpain. J Biol Chem 272, 32719-22 (1997). Pubmed abstract # 9407041
- Chan, K. T., Bennin, D. A. & Huttenlocher, A. Regulation of adhesion dynamics by calpain-mediated proteolysis of focal adhesion kinase (FAK). J Biol Chem 285, 11418-26 (2010). Pubmed abstract # 20150423
- Ridley, A. J. & Hall, A. The small GTP-binding protein rho regulates the assembly of focal adhesions and actin stress fibers in response to growth factors. Cell 70, 389-99 (1992). Pubmed abstract # 1643657
- Amano, M. et al. Phosphorylation and activation of myosin by Rho-associated kinase (Rho-kinase). J Biol Chem 271, 20246-9 (1996). Pubmed abstract # 8702756
- Kimura, K. et al. Regulation of myosin phosphatase by Rho and Rho-associated kinase (Rho-kinase). Science 273, 245-8 (1996). Pubmed abstract # 8662509
- Campellone, K. G. & Welch, M. D. A nucleator arms race: cellular control of actin assembly. Nat Rev Mol Cell Biol 11, 237-51 (2010). Pubmed abstract # 20237478
- Watanabe, N. et al. p140mDia, a mammalian homolog of Drosophila diaphanous, is a target protein for Rho small GTPase and is a ligand for profilin. Embo J 16, 3044-56 (1997). Pubmed abstract # 9214622
- Watanabe, N., Kato, T., Fujita, A., Ishizaki, T. & Narumiya, S. Cooperation between mDia1 and ROCK in Rho-induced actin reorganization. Nat Cell Biol 1, 136-43 (1999). Pubmed abstract # 10559899
- Wen, Y. et al. EB1 and APC bind to mDia to stabilize microtubules downstream of Rho and promote cell migration. Nat Cell Biol 6, 820-30 (2004). Pubmed abstract # 15311282
- Yamashiro, S. et al. Citron kinase, a Rho-dependent kinase, induces di-phosphorylation of regulatory light chain of myosin II. Mol Biol Cell 14, 1745-56 (2003). Pubmed abstract # 12802051
- Ridley, A. J., Paterson, H. F., Johnston, C. L., Diekmann, D. & Hall, A. The small GTP-binding protein rac regulates growth factor-induced membrane ruffling. Cell 70, 401-10 (1992). Pubmed abstract # 1643658
- Bokoch, G. M. Biology of the p21-activated kinases. Annu Rev Biochem 72, 743-81 (2003). Pubmed abstract # 12676796
- Sanders, L. C., Matsumura, F., Bokoch, G. M. & de Lanerolle, P. Inhibition of myosin light chain kinase by p21-activated kinase. Science 283, 2083-5 (1999). Pubmed abstract # 10092231
- Chew, T. L., Masaracchia, R. A., Goeckeler, Z. M. & Wysolmerski, R. B. Phosphorylation of non-muscle myosin II regulatory light chain by p21-activated kinase (gamma-PAK). J Muscle Res Cell Motil 19, 839-54 (1998). Pubmed abstract # 10047984
- Yang, N. et al. Cofilin phosphorylation by LIM-kinase 1 and its role in Rac-mediated actin reorganization. Nature 393, 809-12 (1998). Pubmed abstract # 9655398
- Wittmann, T., Bokoch, G. M. & Waterman-Storer, C. M. Regulation of microtubule destabilizing activity of Op18/stathmin downstream of Rac1. J Biol Chem 279, 6196-203 (2004). Pubmed abstract # 14645234
- Machesky, L. M. & Hall, A. Role of actin polymerization and adhesion to extracellular matrix in Rac- and Rho-induced cytoskeletal reorganization. J Cell Biol 138, 913-26 (1997). Pubmed abstract # 9265656
- Machesky, L. M. et al. Scar, a WASp-related protein, activates nucleation of actin filaments by the Arp2/3 complex. Proc Natl Acad Sci U S A 96, 3739-44 (1999). Pubmed abstract # 10097107
- Nobes, C. D. & Hall, A. Rho, Rac, and Cdc42 GTPases regulate the assembly of multimolecular focal complexes associated with actin stress fibers, lamellipodia, and filopodia. Cell 81, 53-62 (1995). Pubmed abstract # 7536630
- Manser, E., Leung, T., Salihuddin, H., Zhao, Z. S. & Lim, L. A brain serine/threonine protein kinase activated by Cdc42 and Rac1. Nature 367, 40-6 (1994). Pubmed abstract # 8107774
- Symons, M. et al. Wiskott-Aldrich syndrome protein, a novel effector for the GTPase CDC42Hs, is implicated in actin polymerization. Cell 84, 723-34 (1996). Pubmed abstract # 8625410
- Machesky, L. M. & Insall, R. H. Scar1 and the related Wiskott-Aldrich syndrome protein, WASP, regulate the actin cytoskeleton through the Arp2/3 complex. Curr Biol 8, 1347-56 (1998). Pubmed abstract # 9889097
- Osman, M. A. & Cerione, R. A. Iqg1p, a yeast homologue of the mammalian IQGAPs, mediates cdc42p effects on the actin cytoskeleton. J Cell Biol 142, 443-55 (1998). Pubmed abstract # 9679143
- Leung, T., Chen, X. Q., Tan, I., Manser, E. & Lim, L. Myotonic dystrophy kinase-related Cdc42-binding kinase acts as a Cdc42 effector in promoting cytoskeletal reorganization. Mol Cell Biol 18, 130-40 (1998). Pubmed abstract # 9418861
- Etienne-Manneville, S. & Hall, A. Cdc42 regulates GSK-3beta and adenomatous polyposis coli to control cell polarity. Nature 421, 753-6 (2003). Pubmed abstract # 12610628